Quantum computing and supercomputers will revolutionise technology
Quantum computing is an emerging field of study that uses the principles of quantum mechanics to revolutionize the way computers process and store information. Unlike classical computers, which rely on bits to represent data and make changes, quantum computers use quantum bits, or qubits, which can exist in multiple states simultaneously due to a property called superposition.
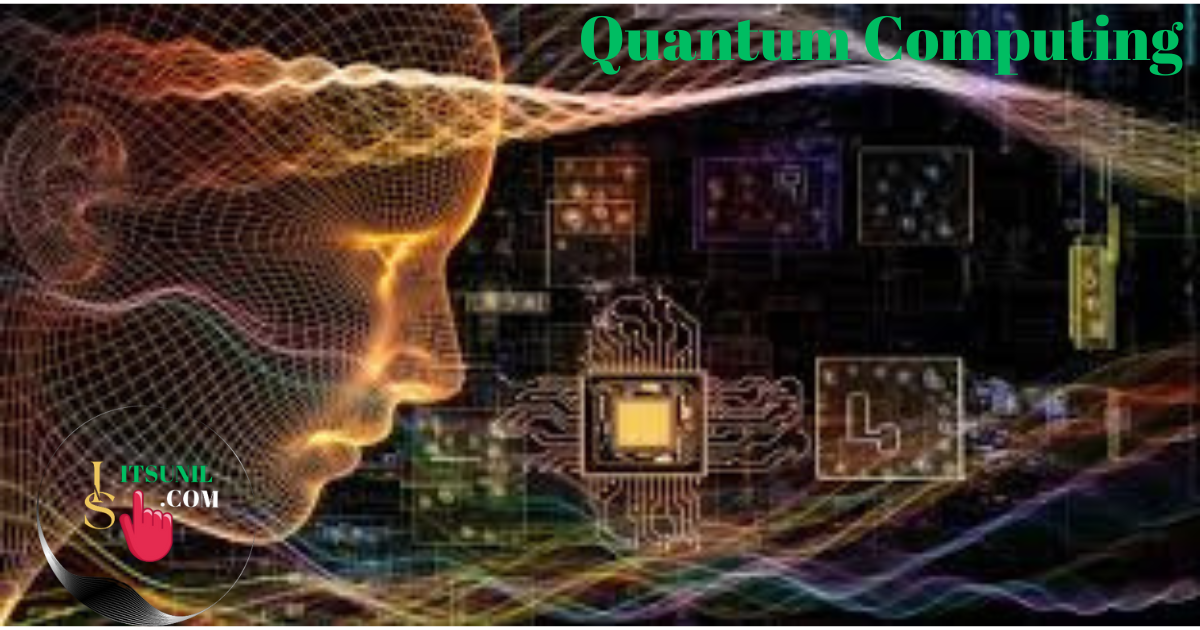
Classical computers work with binary code, where each bit represents either a 0 or a 1. This binary system forms the basis of all digital computing. Quantum computers, on the other hand, use fundamental principles of quantum mechanics, such as superposition and entanglement, to perform calculations in a completely different way.
Superposition allows a qubit to exist in multiple states at the same time. This means that a qubit can represent 0 and 1 simultaneously, instead of being restricted to a single position like a classical bit. This ability to exist in multiple states at once greatly increases the computational power of quantum computers. For example, with just 50 qubits, a quantum computer could potentially store and process 2^50 pieces of information, about a quarter of the number of transistors in today’s most powerful classical computers.
Entanglement is another key feature of quantum computing. When qubits are entangled, the position of one qubit becomes dependent on the position of another, regardless of the distance between them. This phenomenon enables the creation of highly interconnected systems of qubits, allowing quantum computers to perform complex calculations more efficiently than classical computers.
Quantum computing has the potential to solve complex problems that are nearly impossible for classical computers. A good example is factoring, the process of breaking down a large number into its prime factors. Classical computers struggle with this task, especially when the numbers are large enough, but quantum algorithms like Shor’s algorithm can solve factorization problems faster.
Other areas where quantum computing shows promise include optimization problems, quantum system simulation, and cryptography. Quantum computers could significantly improve our ability to optimize processes in various industries, such as logistics, finance, and drug discovery. Furthermore, they can simulate chemical reactions and materials as quantum systems with unprecedented precision and speed, potentially leading to breakthroughs in materials science and drug design.
Despite its immense potential, quantum computing still faces many challenges. One of the most important limitations is the delicate nature of the qubit, which is very sensitive to environmental noise and interference. Researchers are actively exploring various qubit technologies, such as superconducting circuits, trapped ions, and topological qubits, to overcome these challenges and build stable and scalable quantum computers.
Significant advances have been made in the development of quantum computers in recent years. Tech giants, research institutes, and startups are investing heavily in quantum research and development, striving to build reliable quantum computers that can tackle real-world problems. However, it is important to note that quantum computers are still in their infancy, and many technical and practical challenges need to be resolved before they can become mainstream.
Difference between quantum and conventional computing
Quantum computing and traditional computing (classical computing) differ in several key aspects, including their underlying principles, architecture, data representation, and computational capabilities. These are the main differences between quantum and conventional computing:
Basis of Operation
Traditional computing: Classical computers work on the principles of classical physics and use binary digits or bits as the fundamental unit of information. Bits can exist in one of two states: 0 or 1.
Quantum Computing: Quantum computers use the principles of quantum mechanics. They work with quantum bits, or qubits, which can exist in multiple states at once due to superposition. Qubits can represent both 0 and 1 simultaneously, thereby expanding computational
Possibilities:
Traditional computing: Classical computers process data in binary form, where each bit represents a 0 or a 1. Data is manipulated by logic gates that perform specific operations on binary inputs.
Quantum computing: Quantum computers use quantum states to represent and manipulate information. Qubits can represent combinations of 0’s and 1’s through superposition, allowing parallel processing and complex calculations.
Compute Power:
Traditional computing: Classical computers excel at solving many everyday computing tasks, such as arithmetic calculations, data storage, and logical operations. They follow deterministic algorithms and execute instructions sequentially.
Quantum Computing: Quantum computers provide the potential for significant computational advantages for certain problems. They can perform complex calculations faster than classical computers for tasks such as factoring, optimizing and simulating quantum systems. Quantum algorithms can take advantage of superposition and entanglement to provide speedup in specific problem domains.
Bug and noise solution:
Traditional Computing: Classical computers are built with redundancy and error correction mechanisms to reduce errors in computation. Errors are usually caused by hardware failure or transient disturbances.
Quantum computing: Quantum systems are more prone to errors due to environmental noise and interference. Qubits are very sensitive and can quickly lose decoherence. Quantum error correction techniques and fault-tolerant designs are being explored to reduce errors and improve the reliability of quantum computations.
Scalability:
Traditional computing: Classical computers have a well-established technology roadmap for expanding computing power. Increasing performance often means adding more transistors or upgrading hardware components.
Quantum computing: Quantum computers face significant challenges in scaling up due to the delicate nature of qubits and the need to maintain quantum coherence over large numbers of qubits. The development of stable and scalable qubit architectures is a focus of ongoing research.
Current status of development:
Classical computing: Classical computing is a mature field with decades of research, development, and practical applications. It is the foundation of virtually all modern technology and infrastructure.
Quantum computing: Quantum computing is still in its infancy, with research and development continuing. Quantum computers with a limited number of qubits have been built and demonstrated, but practical large-scale quantum computers are still being sought.
In summary, quantum computing differs from conventional computing in its underlying principles, data representation, computational power, error correction, scalability, and current state of development. While classical computers excel at many everyday computing tasks, quantum computers promise to provide significant speed and computational advantages for specific problems, although technical and practical challenges still need to be overcome to reach their full potential. Needed.
Main uses of Quantum Computing
Quantum computing has the potential to revolutionize various fields and solve complex problems that are currently beyond the reach of classical computers. While quantum computers are still in the early stages of development, researchers are discovering a number of promising applications. These are some of the main uses of quantum computing:
Cryptography and Security:
Quantum computers could potentially break many cryptographic algorithms that currently protect sensitive information, such as RSA and elliptic curve cryptography. However, quantum computing may also offer solutions in this area. Quantum cryptography, based on the principles of quantum mechanics, provides secure communication channels by taking advantage of the properties of entanglement and quantum key distribution (QKD). Quantum-resistant encryption algorithms are also being developed to resist attacks from quantum computers.
Optimization and Machine Learning:
Quantum computing has the potential to significantly improve optimization problems arising in various industries such as logistics, supply chain management, finance, and transportation. Quantum algorithms can search large solution spaces more efficiently, leading to better resource allocation, path optimization, portfolio optimization, and more. Quantum machine learning algorithms also hold promise for analyzing large data sets and training complex models, potentially unlocking new insights and improving pattern recognition capabilities.
Simulating Quantum Systems:
Quantum computers excel at simulating quantum systems, which are difficult for classical computers to model accurately. Quantum simulations can provide insight into complex quantum phenomena, physical properties, chemical reactions and molecular dynamics. This could have profound implications for drug discovery, materials science and the development of new materials with unique properties.
Molecular modeling and drug discovery:
Quantum computing can help in the discovery and design of new drugs by simulating molecular interactions with great precision. Quantum algorithms can analyze and optimize molecular structures, predict chemical properties and simulate molecular dynamics more efficiently than classical methods. This capability could significantly speed up the drug discovery process and spur the development of new treatments for a variety of diseases.
Financial Modeling and Risk Analysis:
Quantum computing has the potential to improve financial modeling and risk analysis by enabling faster and more accurate simulations of complex financial systems. You can improve on portfolio optimization, risk assessment, option pricing and algorithmic trading strategies. Quantum algorithms can address complex financial models and optimize investment strategies, leading to more efficient markets and better risk management.
Particle Physics and Cosmology:
Quantum computing can contribute to advances in fundamental physics and cosmology. Quantum algorithms can help simulate particle interactions, analyze large data sets from particle accelerators such as the Large Hadron Collider (LHC), and provide insight into particle behavior at the quantum level. Furthermore, quantum computers can help understand the origin of the universe and solve complex cosmological problems.
It is important to note that although these potential applications are promising, quantum computing is still an emerging field and many technical and practical challenges need to be resolved before it can be widely adopted. However, ongoing research, development, and collaboration among scientists, engineers, and industry leaders is making progress toward realizing the potential of quantum computing in these and other areas.
Future of Quantum Computing
The future of quantum computing holds immense potential for transformative advances in various fields. Although still in its early stages, quantum computing is a rapidly developing field that continues to attract significant attention and investment from researchers, industry leaders and governments around the world. Here are some key aspects shaping the future of quantum computing:
Scalability and stability: One of the main challenges of quantum computing is to achieve scalability while maintaining the stability of qubits. Researchers are exploring various qubit technologies, such as superconducting circuits, trapped ions, topological qubits, and more, to improve qubit stability, coherence time, and error rates. Advances in qubit design, error-correction techniques, and fault-tolerant architectures are essential to building reliable, large-scale quantum computers.
Quantum supremacy: Quantum supremacy refers to the milestone at which a quantum computer can perform calculations that exceed the capabilities of even the most powerful classical computers. Achieving quantum supremacy would validate the potential of quantum computing and show its superiority for specific tasks. While quantum supremacy has been proven for some problems, future advances in hardware, algorithms and error correction will push the limits of what quantum computers can achieve.
Hybrid approach: Hybrid quantum-classical algorithms combine the powers of classical and quantum computing to tackle complex problems. These approaches harness the computational power of quantum computers for specific subtasks, while using classical computers for pre- and post-processing. Hybrid algorithms bridge the gap between classical and quantum computing and are expected to play an important role in the near future, especially as quantum computers continue to develop.
Quantum software and programming languages: As quantum computers advance, there is a growing need for specialized quantum software and programming languages. Efforts are underway to develop quantum programming languages, frameworks and software tools that make it easier for researchers and developers to write and optimize quantum algorithms. These advances will allow the wider community to effectively access and use quantum computers.
Real-world applications and implications: Quantum computing has the potential to revolutionize various industries and solve complex problems. As quantum hardware and algorithms mature, we can expect significant advances in cryptography, optimization, materials science, drug discovery, machine learning, finance, and other fields. Quantum computers will provide novel insights, speed up calculations and open up solutions to challenges that are currently beyond the capabilities of classical computers.
Quantum Internet and Quantum Communication: The development of a quantum Internet is an active area of research. Quantum communication protocols and quantum networks can enable the secure and efficient transmission of quantum information over long distances. Quantum networks will facilitate quantum key distribution (QKD), quantum teleportation and distributed quantum computing, enabling secure and collaborative quantum computing across multiple locations.
Collaboration and Interdisciplinary Research: Quantum computing requires collaboration between scientists, engineers, mathematicians, physicists, computer scientists, and industry experts. Interdisciplinary research and knowledge sharing will drive advances in quantum technologies, algorithms and applications. Collaborative efforts among academia, industry, and governments are necessary to accelerate progress and overcome the challenges associated with quantum computing.
The future of quantum computing has tremendous potential to transform various industries, revolutionize computational capabilities, and tackle complex problems. Continued research, development and investment will drive the progress of quantum technologies, broaden their impact and pave the way for practical and scalable quantum computers that can solve real-world challenges for years to come.
Conclusion
In conclusion, quantum computing is a rapidly developing field that uses the principles of quantum mechanics to process and store information. By harnessing the power of superposition and entanglement, quantum computers have the potential to solve complex problems that are currently beyond the reach of classical computers. While significant challenges remain to be overcome, advances in quantum computing promise to transform various industries and revolutionize the way problems are solved in the future.
FAQs
Question: What is quantum computing?
A: Quantum computing is a field that uses the principles of quantum mechanics p
Question: How are qubits different from classical bits?
Answer: Qubits differ from classical bits in that they can exist in multiple states at once due to a property called superposition. Whereas classical bits can represent either 0 or 1, qubits can represent both 0 and 1 simultaneously, which allows for parallel processing and higher computational power.
Q: What is overlap?
A: Superposition is a fundamental principle of quantum mechanics that allows qubits to exist in multiple states at the same time. This allows qubits to represent combinations of 0 and 1, rather than being restricted to a single position like classical bits. This property allows quantum computers to perform calculations in parallel and solve some problems more efficiently.
Question: What is entanglement in quantum computing?
A: Entanglement is another important concept in quantum computing. When qubits are entangled, the position of one qubit becomes correlated with the position of another, regardless of the distance between them. This phenomenon allows quantum computers to create highly interconnected systems of qubits, leading to improved computational capabilities and the potential for more efficient problem solving.
Question: What are some possible applications of quantum computing?
Answer: Quantum computing has the potential to impact many areas. Some potential applications include cryptography, where quantum computers could break conventional encryption algorithms and enable secure quantum communications. Quantum computing can also improve optimization problems, simulate quantum systems for materials science and drug discovery, and improve machine learning algorithms for data analysis.
Question: Are there practical quantum computers available today?
Answer: Practical quantum computers that can solve complex problems at scale are still in the early stages of development. While quantum computers with a limited number of qubits have been built, they have not been able to outperform classical computers for most tasks. However, ongoing research and advances in hardware, software and error correction techniques are bringing us closer to practical quantum computers.
Question: What are the challenges of quantum computing?
Answer: Quantum computing faces many challenges. It is important to maintain the stability and coherence of the qubits, as they are sensitive to environmental noise and interference. Scaling up quantum systems while minimizing errors is another challenge. Developing robust error correction techniques, improving qubit reliability and building scalable architectures are active areas of research in this area.
Question: When will we see the widespread adoption of quantum computers?
Answer: The timeline for the widespread adoption of quantum computers is uncertain. This depends on overcoming technical challenges, improving qubit stability, and developing error correction methods. Although it may take years or even decades, continued progress in research and development is paving the way for practical quantum computers to become a reality in the future.